Nerve Tissue Composition
Nerve tissue is a fundamental component of the nervous system, responsible for transmitting signals throughout the body. Comprising of specialized cells called neurons, nerve tissue is characterized by its unique structure and function.
Table of Contents
What is a Nerve Tissue Composition?
Within the neurological system, neurons are cells that send messages to other neurons, muscles, or gland cells. An axon, dendrites, and a cell body are present in most neurons. The cytoplasm and nucleus are found in the cell body. After emerging from the cell body, the axon frequently splits into numerous smaller branches before coming to an end at nerve terminals.
Neurons send and receive messages through dendrites, which protrude from the cell body of the neuron. The points of contact between neurons where communication occurs are called synapses. The terminals of axons from different neurons form synapses that cover the dendrites.
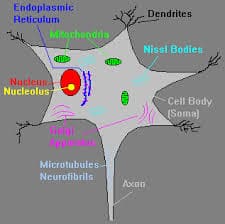
The anatomical and functional characteristics of linked neurons make the brain what it is. Depending on the species, the mammalian brain has anywhere from 100 million to 100 billion neurons. An axon, dendrites, and cell body make up every mammalian neuron.
The cytoplasm and nucleus are found in the cell body. After emerging from the cell body, the axon frequently splits into numerous smaller branches before coming to a halt at nerve terminals.
Neurons send and receive messages through dendrites, which protrude from the cell body of the neuron. The points of contact between neurons where communication occurs are called synapses. The terminals of axons from different neurons form synapses that cover the dendrites.
Electrical impulses are sent down axons, which can be as short as a fraction of an inch (or centimeter) or as long as three feet (about one meter) or longer, by neurons when they receive or send messages. The multilayer myelin coating that covers many axons expedites the passage of electrical signals across the axon. Glia are specialized cells that produce this sheath. The glia that form the sheath are referred to as Schwann cells in the peripheral nervous system and oligodendrocytes in the brain.
Glia are at least ten times more abundant in the brain than neurons. Glia has multiple roles. Glia has long been understood to provide nutrients to neurons, remove waste from the brain, break down sections of dead neurons, and support the integrity of neurons. Important new roles for glia in brain function are being revealed by current research.
Nerve tissue is composed of:
- Water…………80 percent.
- Solids…………20 per cent.
Proteins, fats, trace amounts of organic extracts, and inorganic salts make up the majority of the solids. About 38–40% of the total solids are proteins. These consist of several globulins, nucleoproteins, and neurokeratin, a unique albuminoid. Between 50 and 54 percent of the total solids are made up of lipids.
Phospholipids, cholesterol, cerebrosides, amino-lipids, and lipids containing sulfur are among the significant lipids. Potassium phosphate and chloride are the main inorganic salts; sodium and other alkaline elements are present in lower levels. Potassium has a major role in the nerve impulse.
The brain’s water content is barely higher than the spinal cord’s. The brain’s grey matter, which is a concentration of nerve cell bodies, has a higher water content than the whole matter, which is home to the majority of the nerve fibers. Ceruloplasmin is formed when some brain proteins interact with copper.
In Wilson’s disease, there is an elevated concentration of copper in the brain tissue. Cerebrosides, free cholesterol, and sphingomyelin are slightly higher in white matter and peripheral nerves than in brain grey matter. Niemann-Pick disease is associated with a significant elevation in sphingomyelin content.
Types of Nervous Tissue
Nervous tissue, which makes up the nervous system, is mostly made up of two types of cells: neurons and neuroglia.
Neuroglia also referred to as “glial cells,” are the supporting cells that make up nervous tissue.
Neuroglia are classified into six groups. Two are located in the peripheral nervous system and four in the central nervous system.
Astrocytes, microglial cells, ependymal cells, and oligodendrocytes are the four different forms of neuroglia that are present in the central nervous system.
Schwann cells and satellite cells are the two varieties of neuroglia that are present in the peripheral nervous system.
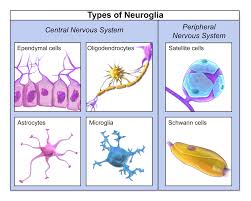
The other type of cell that makes up nervous tissue is called a neuron. Axons, dendrites, and cell bodies are features of neurons.
Neuron
the predominant kind of cell in nerve tissue.
neuroglia: Cells that support nerve tissue.
One of the four primary tissue types is nervous tissue, which is made up of neurons, and neuroglia, which are supporting cells. Another name for neuroglia is “glial cells.”
Neuroglia
Six different forms of neuroglia exist two in the PNS and four in the central nervous system. Other than supporting the neurons, these glial cells have a variety of specific roles. Astrocytes, microglial cells, ependymal cells, and oligodendrocytes are examples of neuroglia in the central nervous system. Schwann cells and satellite cells are the two varieties of neuroglia found in the PNS.
Astrocytes
Astrocytes are the most prevalent glial cells in the central nervous system, and they have a star-like form. Their numerous radiating processes aid in their ability to adhere to capillaries and neurons.
They bind the neurons to the nutrient supply lines and provide support and bracing for the neurons. Additionally, they aid in directing the migration of developing neurons. The chemical environment around neurons is regulated by astrocytes.
Microglial Cells
Microglial cells have thorny processes and are tiny, and ovoid in shape. The CNS contains them. The ability of microglial cells to change into phagocytic macrophages and aid in the removal of neuronal debris is present when there are invasive microorganisms or deceased neurons.
Ependymal Cells
The core cavities of the brain and spinal cord are lined with ciliated endodermal cells, which create a somewhat permeable barrier between the tissue cells of the central nervous system and the cerebrospinal fluid that fills these spaces.
Oligodendrocytes
Along the nerves, oligodendrocytes form the myelin sheath, an insulating covering. The CNS contains them.
Satellite Cells
In the peripheral nervous system, satellite cells encircle the cell bodies of neurons (PNS). They bear a resemblance to the CNS’s astrocytes.
Schwann Cells
In the peripheral nervous system, Schwann cells encircle every nerve fiber and create myelin sheaths surrounding them. The PNS contains them. They serve a similar purpose as oligodendrocytes.
Neurons
A neuron’s cell body and one or more thin processes make up the neuron. The rough endoplasmic reticulum, often known as Nissl bodies, and the nucleus make up the neuronal cell body. The primary site of biosynthesis in a neuron is the cell body, which also houses the typical organelles needed for the creation of various molecules and proteins. All neurons have arm-like processes that extend from the cell body.
Neuron processes can be classified as either dendrites or axons. Short motor neurons with a lot of surface area for receiving messages from neighboring neurons are called dendrites. Dendrites are referred to as the receptive input region because they transmit incoming messages to the cell body.
The cone-shaped area of the cell body known as the axon hillock is where the axon originates. In terms of function, the axon is the conducting section of the neuron and is in charge of producing and sending impulses, usually in the direction of the cell body. The nerve impulse travels by a single axon from the cell body to an effector organ or another neuron. Multiple terminal branches of the axon allow the neuron to fire multiple times, stimulating multiple cells.
Component of Nerve Tissue
Components
Neurons are specialized cells that have the ability to receive and facilitate action potentials, which are nerve impulses, across their membrane to the next neuron. They have an axon and a huge cell body known as the soma, along with cell extensions known as dendrites.
Neurotransmitters, or electrochemical signals, are received via dendrites, which are slender, branching projections that alter the voltage within a cell. Long projections called axons are responsible for transporting the action potential from the cell body to the subsequent neuron. A tiny opening known as a synaptic cleft divides the dendrite of the subsequent neuron from the bulbous end of the axon, which is referred to as the axon terminal.
Neurotransmitters are released across the synapse and attach to the post-synaptic receptors to continue the nerve impulse when the action potential reaches the axon terminal.
There are two categories for neurons: structural and functional.
Functional classification:
Sensory neurons (afferent):
Send a nerve impulse, or action potential, from the PNS to the CNS to convey sensory data.
Motor neurons (efferent):
Send an action potential from the central nervous system to the appropriate effector (muscles, glands).
Interneurons:
Cells in the brain or spinal cord that connect neurons and whose functions are confined to a certain local region
Structural classification:
Multipolar neurons:
has at least three processes originating from the soma (cell body). Interneurons and motor neurons are among the main categories of neurons in the central nervous system.
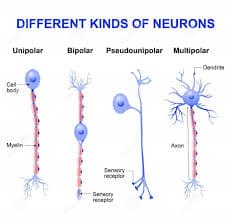
Bipolar neurons:
sensory neurons that have an axon and a dendrite emerging from the soma
Pseudounipolar neurons:
Sensory neurons have a single process that divides into the axon and dendrite.
Unipolar brush cells:
are glutamatergic excitatory interneurons with a single short dendrite that ends in a tuft of dendrioles that resembles a brush. These are situated in the granular layer of the cerebellum.
The non-neural cells in nervous tissue that perform a variety of very important supporting roles for neurons are referred to as neuroglia. Depending on their function, they have different structures and are smaller than neurons.
Neuroglial cells are classified as follows:
Microglial cells:
The macrophage cells known as microglia comprise the central nervous system’s major immune system. The tiniest neuroglial cells are what they are.
Astrocytes:
Macroglial cells in the CNS resemble stars and have numerous functions. They are essential to a healthy central nervous system and are the most prevalent cell type in the brain.
Oligodendrocytes:
neurons in the brain with minimal processes. On a neuron’s axons, they produce myelin sheaths, lipid-based insulation that quickens the action potential’s passage through the axon.
NG2 glia:
CNS cells that function as oligodendrocytes’ developmental progenitors and are different from astrocytes, oligodendrocytes, and microglia
Schwann cells:
They are the PNS counterparts of oligodendrocytes, aiding in the formation of myelin sheaths and axon maintenance.
Satellite glial cell:
Cover the surface of ganglia’s (the PNS’s clusters of nerve body cells coiled or linked together) neuron cell bodies.
Enteric glia: located in the gastrointestinal tract and within the enteric nerve system.
Metabolism of the Nerve Tissue
Because the metabolic nerve’s respiratory quotient (R.Q.) is so close to 1, it suggests that the nerve tissues nearly exclusively use carbohydrates for energy.
The breakdown of carbohydrates in nerve tissue produces lactic and pyruvic acids, which are produced in anaerobic environments and degrade very slowly in the presence of oxygen. As a result, it appears that nerve tissue and muscle tissue have comparable metabolic processes for carbohydrates.
Because there is less glycogen stored in neural tissue, the nervous system depends particularly on a small quantity of blood glucose. This could be the main reason why nervous symptoms like delirium, dizziness, and mental confusion are more common when hypoglycemia is present.
Cross-Section of a Small Nerve Trunk
The sole amino acid that brain tissue metabolizes in terms of protein metabolism is glutamic acid. This acid acts as a significant acceptor of ammonia produced by the brain or given to it when arterial blood ammonia levels are elevated. It also functions as a precursor of -aminobutyric acid (GABA), one of the chemical transmitters, protecting brain tissue from its toxic effects.
Additionally, the creation and exchange of lipids in nerve tissue have been shown by many researchers.
Nerve Impulse:
An electrochemical shift that is carried by nerve fibers is known as a nerve impulse. It should not be mistaken for the stimulus, which is the outside factor (chemical, physical, biological, etc.) that initiates the impulse.
The recuperation mechanisms that ensue after the activity are affected by the chemical alterations in the nerve fibers. The most reliable indicator of the genesis and propagation of a nerve impulse is an electrical shift, which also serves as a representation of the crucial step in the impulse’s passage through the nerve fiber.
Transmission of Nerve Impulse from One Neurone of Other:
A synapse is a point where one neurone joins another, while a synaptic cleft is a small slit that divides successive neurones from one another. A presynaptic terminal and a portion of the perikaryon make up a synapse, and they are divided by a synaptic cleft that is typically 200 Ã… wide.
Physiologic Anatomy of the Synapse
Synaptic vesicles and mitochondria are found in the presynaptic terminal.
The synaptic vesicles contain chemical transmitters that can be either excitatory or inhibitory to the next neuron, in which case the presynaptic terminal is referred to as an inhibitory presynaptic terminal, and the parent neurone is referred to as an inhibitory neurone.
These vesicles release transmitter material into the synaptic cleft when the action potential reaches the presynaptic terminal. When the transmitter is excitatory, it immediately increases the permeability of the subsynaptic somal membrane to either Na+ or K+. When it is inhibitory, it does the opposite.
Na+ reaches the interior of the perikaryon of the subsequent neurone when its permeability rises. This excites this neurone by causing a series of modifications that culminate in an action potential known as the excitatory post-synaptic potential (EPSP).
However, if the release of an inhibitory transmitter causes the permeability to increase to K+, the excess K+ within the cell causes K to flow out of the membrane quickly, leaving a higher level of negativity inside the neurone.
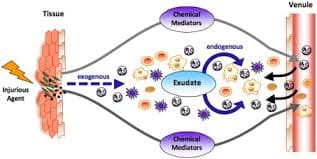
Because it inhibits the following neuron or effector cell, this condition is referred to as hyper-polarization, and the potential resulting from it is called the Inhibitory Postsynaptic Potential (IPSP). Because chemical transmitters are involved in the aforementioned process, it is also known as the chemical transmission of nerve impulses.
Nature of the Chemical Transmitters Released by Nerve Tissue:
In 1921, Loewi made the initial discovery of the chemical transmission method. He put the fluid from a perfused frog heart into another heart and demonstrated that this fluid similarly produced the usual vagal effects of depression in the second heart when the vagus nerve supplying the first heart was activated.
In a similar vein, stimulating the first heart’s sympathetic nerve supply likewise stimulated the second heart. The material so released by vagus stimulation was referred to by Loewi as “vagusstoff,” and the substance released by sympathetic stimulation as “acceleranstoff.” Subsequent research revealed that these substances were noradrenaline and acetylcholine, respectively.
For a substance to function as a transmitter, it must meet the following requirements:
a. The substance (the transmitter) must be released from the nerve terminals during nerve stimulation.
b. Substances that oppose a substance’s action at the postsynaptic membrane ought to obstruct the consequences of the nerve stimulation that substance transmits as well.
c. Chemicals (drugs) that prevent this substance from synthesizing or being stored should prevent this substance’s effects on nerve stimulation from being conveyed.
d. A method for destroying or eliminating the suggested transmitter is required. For example, the enzyme acetyl cholinesterase is required to destroy acetyl choline, and neurons that use acetyl choline as their transmitter must contain this enzyme.
g. It needs to be produced and kept in neurones.
f. If a material is supplied from the outside, its effects on the innervated organ must be equivalent to those on the stimulation of the nerves that release it.
g. Drugs that alter the removal or destruction of transmitters must also appropriately alter the body’s reaction to nerve stimulation.
Acetylcholine, adrenaline, and norepinephrine are the transmitters that meet the aforementioned requirements.
Who are the potential transmitters?
Dopamine, glutamic acid, glycine, serotonin, and gamma-aminobutyric acid (GABA).
Cholinergic and Adrenergic Nerve Fibres:
According to Dale, cholinergic fibers are nerve fibers that form and release acetylcholine as a transmitter, whereas adrenergic fibers are nerve fibers that form and release noradrenaline.
Cholinergic nerve fibres are as follows:
- a. Every pre-ganglionic fiber in the sympathetic and parasympathetic nervous systems.
- b. Every parasympathetic system post-ganglionic fiber.
- c. A few post-ganglionic sympathetic nerve fibers, such as nerve fibers that supply the sweat glands.
- d. The skeletal muscles’ motor nerve fibers.
- d. A portion of the CNS’s neurons.
- f. Efferent fibers supplying the adrenal medullary gland.
The adrenergic fibres are as follows:
- The majority of the sympathetic system’s post-ganglionic fibers.
- A portion of the central nervous system’s neurons.
The process by which an impulse travels from a nerve to a skeletal muscle:
A neuromuscular junction is defined as the junction of a motor nerve fiber and a skeletal muscle fiber. A motor unit consists of a motor neurone and the collection of muscle fibers it innervates.
Neruromuscular Junction and Invagination of a Sole
The myelinated nerve fiber loses its medullary sheath as it gets closer to a skeletal muscle fiber, and at its terminal end, it forks into terminal ramifications. The true neuromuscular junction is formed at the terminals of these terminal branches of the nerve fiber by additional structure known as sole feet, which make a thorough contact with a specific area of the muscle fiber membrane.
The motor end plate is the portion of the muscle fiber membrane that makes contact with the sole of the foot. It is folded into several folds, increasing the amount of surface area available for action by the synaptic transmitter.
Around the edge of the motor end plate are big clumps of the enzyme cholinesterase, which can break down acetylcholine, the synaptic transmitter.
The synaptic cleft, which divides the sole foot from the synaptic gutter (motor end plate), is filled with a gelatinous “ground substance” that allows extracellular fluid to permeate through. Numerous tiny vesicles found in the sole foot store the synaptic transmitter, which is most likely produced by mitochondria that also provide the energy needed for synthesis.
The sequence of events that take place at the neuromuscular junction during the transmission of a nerve impulse from a motor nerve fiber to a skeletal muscle fiber can be discussed as follows, following the description of the structural characteristics of the neuromuscular junction:
A. The moment an impulse reaches the terminal motor nerve.
B. Acetylcholine-containing synaptic vesicles are ruptured by calcium ions (Ca++) via the sole foot membrane, releasing acetylcholine into the synaptic cleft where it binds to end plate receptors (cholinergic receptors). The release of acetylcholine is blocked by magnesium ions (Mg++).
C. Hypothetical Ca++ migrations from the extracellular fluid into the sole foot membrane.
D. The propagation of the end-plate potential so generated upon reaching a threshold, or a critical magnitude.
e. An increase in the muscle membrane’s permeability to Na+ causes a quick influx of Na+ into the muscle fiber, producing the local end-plate potential, a potential that is exactly like the excitatory post-synaptic potential.
f. Within two milliseconds, acetylcholine is removed from its receptor location, partially through cholinesterase breakdown and partially through diffusion back into the sole foot.
Processes Involved in Synthesis, Release, and Disposal of Acetyhlcholine
The amount of acetylcholine released with each impulse decreases when the nerve fiber is stimulated at a pace faster than 150 times per second for several minutes; impulses may even fail to enter the muscle fiber.
It is known as the neuromuscular junction fatigue and is comparable to synaptic fatigue. However, since even the most active neuromuscular junctions get more than 150 impulses per second, fatigue of the neuromuscular junction never happens under normal circumstances.
Classification of Nerve Tissue:
In the central nervous system:
Cell bodies, dendrites, unmyelinated axons, satellite oligodendrocytes (a non-myelinating oligodendrocyte subtype), protoplasmic astrocytes (an astrocyte subtype), microglia, and a negligible number of myelinated axons make up grey matter.
Myelinating oligodendrocytes, fibrous astrocytes, myelinated axons, and microglia make up white matter.
In the peripheral nervous system
Satellite glial cells, dendrites, and cell bodies make up ganglion tissue.
Schwann cells encased in connective tissue, both myelinated and unmyelinated, make up nerves.
Each nerve is surrounded by three layers of connective tissue, which are:
Endoneurium
The endoneurium, also known as the endoneurial tube, channel, or sheath, envelops each nerve fiber or axon. This layer of connective tissue is thin, sensitive, and protective.
Perineurium
The perineurium, a connective tissue consisting of seven or eight concentric layers, encloses each nerve fascicle that contains one or more axons. This is crucial for maintaining and safeguarding the nerve fibers as well as preventing big molecules from entering a fascicle from the epineurium.
Epineurium
The outermost layer of the (peripheral) nerve’s dense connective tissue is called the epineurium.