MUSCULAR SYSTEM
Table of Contents
What is a Muscular System?
- First, let’s take a brief look at the muscles themselves. Like so many other things, muscles are made up of lots of smaller parts. We’ll start at the level of the muscle fiber. A muscle fiber is simply a muscle cell, or the building blocks of muscles. See, just like the rest of your body is made up of lots and lots of individual cells, your muscles are also made up of cells.
- Multiple fibers join together to make up our next level of organization, the fascicle. A fascicle is a group, or bundle, of muscle fibers. And, just like many muscle fibers join together to form a fascicle, many fascicles join together to make up a muscle.
- Muscles are composed of fibers and fascicles.
- Components of Muscles
- Muscles are made up of groups of fascicles. When stimulated, they contract to produce motion.
Without muscles, your body wouldn’t be able to function. No more running, talking, walking and, well.
- The organization of the muscle allows all the fibers, and thus the fascicles, to contract and relax as a group. Contraction is stimulated by nerve impulses and triggers the movement of the muscle, while relaxation occurs when the impulse is removed and the muscle relaxes back to its natural state. This pattern of contraction and relaxation is responsible for all the movements in your body.
- The part of your body that moves in response to a muscle contraction depends on the location and origin point of the muscles themselves. To simplify things, we are going to focus on the skeletal muscles of the body. Most skeletal muscles are attached to bone, cartilage, or connective tissue, which limits or directs their movement. For example, a muscle attached to the arm bone will only move the arm bone when stimulated. It cannot move the leg bone; therefore, its movement is determined by its points of attachment.
- the muscular system
- it probably seems overwhelming at first, what with all those funky scientific names. There are over 600 skeletal muscles, and that number doesn’t even include the smooth muscles! But, like everything else in your body, the muscular system has its own sense of organization. Once you learn a little bit about how it works, it may begin to make a little more sense to you.
- Even the naming system is organized. Some muscles are named for their location, while others are named for their shape or the direction in which their fibers run. We won’t get into all those names in this lesson, but we will get started with some of the basics. As you have probably guessed, the main function of the muscular system is movement, but it also helps stabilize our joints, maintain our posture and generate heat during activity.
- Movement of our body can be voluntary and controlled by the skeletal muscles, or it can be involuntary and controlled by smooth muscles. Those are found mostly in your internal organs and aid in things like digestion and even eyesight. Skeletal muscles, on the other hand, are what make up what we see a human body do. They move all the different parts of the skeleton. They help us in all kinds of movements, such as walking, swimming, writing and even talking. All this movement is aided by the anatomy and organization of the muscular system. But, before we move on, we should mention you do have one other type of muscle in your body. Cardiac muscles are found in the heart and are responsible for the movement of blood through your body.
Origin and Insertion Points
- Each muscle has two main points of attachment, the origin point and the insertion point. The origin of a muscle is the fixed end, the end that doesn’t move. This end is usually a bone, cartilage or connective tissue. The opposite end of the muscle is the insertion point. This is the movable end of the muscle which is attached to the structure that is being moved.
- The origin and insertion points of the biceps brachii
Origin Insertion Point Diagram
Let’s go back to our arm muscle example. If we take a look at the upper arm bone, called the humerus,
we can see a large muscle on top of it. This muscle, called the biceps brachii has a point of origin
at the scapula, and its insertion point on the radius of the lower arm. When the muscle contracts, it
moves the radius upwards towards the scapula, but the scapula and upper arm do not move. That is why
the radius is termed the insertion point, while the scapula is the origin point. - If you’re still a little confused about how to tell the difference, you can give it a try on your
own. When you move your arm or your leg, take notice of which end of the muscle is doing the moving
and which end is staying still. That will help you figure out which is the insertion (that’s the one
doing the moving) and which is the origin.
- There are three types of muscle tissue: Visceral, cardiac, and skeletal.
- Visceral Muscle:
Visceral muscle is found inside of organs like the stomach, intestines, and blood vessels. The weakest of all muscle tissues, visceral muscle makes organs contract to move substances through the organ. Because visceral muscle is controlled by the unconscious part of the brain, it is known as involuntary muscle—it cannot be directly controlled by the conscious mind. The term “smooth muscle” is often used to describe visceral muscle because it has a very smooth, uniform appearance when viewed under a microscope. This smooth appearance starkly contrasts with the banded appearance of cardiac and skeletal muscles.
- Cardiac Muscle:
Found only in the heart, cardiac muscle is responsible for pumping blood throughout the body. Cardiac muscle tissue cannot be controlled consciously, so it is an involuntary muscle. While hormones and signals from the brain adjust the rate of contraction, cardiac muscle stimulates itself to contract. The natural pacemaker of the heart is made of cardiac muscle tissue that stimulates other cardiac muscle cells to contract. Because of its self-stimulation, cardiac muscle is considered to be autorhythmic or intrinsically controlled.
The cells of cardiac muscle tissue are striated—that is, they appear to have light and dark stripes when viewed under a light microscope. The arrangement of protein fibers inside of the cells causes these light and dark bands. Striations indicate that a muscle cell is very strong, unlike visceral muscles.
The cells of cardiac muscle are branched X or Y-shaped cells tightly connected together by special junctions called intercalated disks. Intercalated disks are made up of fingerlike projections from two neighboring cells that interlock and provide a strong bond between the cells. The branched structure and intercalated disks allow the muscle cells to resist high blood pressures and the strain of pumping blood throughout a lifetime. These features also help to spread electrochemical signals quickly from cell to cell so that the heart can beat as a unit.
- Skeletal Muscle:
Skeletal muscle is the only voluntary muscle tissue in the human body—it is controlled consciously. Every physical action that a person consciously performs (e.g. speaking, walking, or writing) requires skeletal muscle. The function of skeletal muscle is to contract to move parts of the body closer to the bone that the muscle is attached to. Most skeletal muscles are attached to two bones across a joint, so the muscle serves to move parts of those bones closer to each other.
Skeletal muscle cells form when many smaller progenitor cells lump themselves together to form long, straight, multinucleated fibers. Striated just like cardiac muscle, these skeletal muscle fibers are very strong. Skeletal muscle derives its name from the fact that these muscles always connect to the skeleton in at least one place.
- Gross Anatomy of a Skeletal Muscle
Most skeletal muscles are attached to two bones through tendons. Tendons are tough bands of dense regular connective tissue whose strong collagen fibers firmly attach muscles to bones. Tendons are under extreme stress when muscles pull on them, so they are very strong and are woven into the coverings of both muscles and bones.
Muscles move by shortening their length, pulling on tendons, and moving bones closer to each other. One of the bones is pulled towards the other bone, which remains stationary. The place on the stationary bone that is connected via tendons to the muscle is called the origin. The place on the moving bone that is connected to the muscle via tendons is called the insertion. The belly of the muscle is the fleshy part of the muscle in between the tendons that does the actual contraction.
- Names of Skeletal Muscles
Skeletal muscles are named based on many different factors, including their location, origin and insertion, number of origins, shape, size, direction, and function.
Location.
Many muscles derive their names from their anatomical region. The rectus abdominis and transverse abdominis, for example, are found in the abdominal region. Some muscles, like the tibialis anterior, are named after the part of the bone (the anterior portion of the tibia) that they are attached to. Other muscles use a hybrid of these two, like the brachioradialis, which is named after a region (brachial) and a bone (radius).
- Origin and Insertion. Some muscles are named based upon their connection to a stationary bone (origin) and a moving bone (insertion). These muscles become very easy to identify once you know the names of the bones that they are attached to. Examples of this type of muscle include the sternocleidomastoid (connecting the sternum and clavicle to the mastoid process of the skull) and the occipitofrontalis (connecting the occipital bone to the frontal bone).
- Number of Origins. Some muscles connect to more than one bone or to more than one place on a bone, and therefore have more than one origin. A muscle with two origins is called a biceps. A muscle with three origins is a triceps muscle. Finally, a muscle with four origins is a quadriceps muscle.
- Shape, Size, and Direction. We also classify muscles by their shapes. For example, the deltoids have a delta or triangular shape. The serratus muscles feature a serrated or saw-like shape. The rhomboid major is a rhombus or diamond shape. The size of the muscle can be used to distinguish between two muscles found in the same region. The gluteal region contains three muscles differentiated by size—the gluteus maximus (large), gluteus medius (medium), and gluteus minimus (smallest). Finally, the direction in which the muscle fibers run can be used to identify a muscle. In the abdominal region, there are several sets of wide, flat muscles. The muscles whose fibers run straight up and down are the rectus abdominis, the ones running transversely (left to right) are the transverse abdominis, and the ones running at an angle are the obliques.
- Function.
Muscles are sometimes classified by the type of function that they perform. Most of the muscles of the forearms are named based on their function because they are located in the same region and have similar shapes and sizes. For example, the flexor group of the forearm flexes the wrist and the fingers. The supinator is a muscle that supinates the wrist by rolling it over to face palm up. In the leg, there are muscles called adductors whose role is to adduct (pull together) the legs.
Groups Action in Skeletal Muscle :
- Skeletal muscles rarely work by themselves to achieve movements in the body. More often they work in groups to produce precise movements. The muscle that produces any particular movement of the body is known as an agonist or prime mover. The agonist always pairs with an antagonist muscle that produces the opposite effect on the same bones. For example, the biceps brachii muscle flexes the arm at the elbow. As the antagonist for this motion, the triceps brachii muscle extends the arm at the elbow. When the triceps is extending the arm, the biceps would be considered the antagonist.
- In addition to the agonist/antagonist pairing, other muscles work to support the movements of the agonist. Synergists are muscles that help to stabilize a movement and reduce extraneous movements. They are usually found in regions near the agonist and often connect to the same bones. Because skeletal muscles move the insertion closer to the immobile origin, fixator muscles assist in movement by holding the origin stable. If you lift something heavy with your arms, fixators in the trunk region hold your body upright and immobile so that you maintain your balance while lifting.
Skeletal Muscle Histology :
- Skeletal muscle fibers differ dramatically from other tissues of the body due to their highly specialized functions. Many of the organelles that make up muscle fibers are unique to this type of cell.
- The sarcolemma is the cell membrane of muscle fibers. The sarcolemma acts as a conductor for electrochemical signals that stimulate muscle cells. Connected to the sarcolemma are transverse tubules (T-tubules) that help carry these electrochemical signals into the middle of the muscle fiber. The sarcoplasmic reticulum serves as a storage facility for calcium ions (Ca2+) that are vital to muscle contraction. Mitochondria, the “power houses” of the cell, are abundant in muscle cells to break down sugars and provide energy in the form of ATP to active muscles. Most of the muscle fiber’s structure is made up of myofibrils, which are the contractile structures of the cell. Myofibrils are made up of many proteins fibers arranged into repeating subunits called sarcomeres. The sarcomere is the functional unit of muscle fibers. (See Macronutrients for more information about the roles of sugars and proteins.)
- Sarcomere Structure
Sarcomeres are made of two types of protein fibers: thick filaments and thin filaments.
1)Thick filaments. Thick filaments are made of many bonded units of the protein myosin. Myosin is the protein that causes muscles to contract.
2) Thin filaments. Thin filaments are made of three proteins:
Actin. Actin forms a helical structure that makes up the bulk of the thin filament mass. Actin contains myosin-binding sites that allow myosin to connect to and move actin during muscle contraction.
Tropomyosin. Tropomyosin is a long protein fiber that wraps around actin and covers the myosin binding sites on actin.
Troponin. Bound very tightly to tropomyosin, troponin moves tropomyosin away from myosin binding sites during muscle contraction.
Muscular System Physiology :
- The function of Muscle Tissue
The main function of the muscular system is movement. Muscles are the only tissue in the body that has the ability to contract and therefore move the other parts of the body.
- Related to the function of movement is the muscular system’s second function: the maintenance of posture and body position. Muscles often contract to hold the body still or in a particular position rather than to cause movement. The muscles responsible for the body’s posture have the greatest endurance of all muscles in the body—they hold up the body throughout the day without becoming tired.
- Another function related to movement is the movement of substances inside the body. The cardiac and visceral muscles are primarily responsible for transporting substances like blood or food from one part of the body to another.
- The final function of muscle tissue is the generation of body heat. As a result of the high metabolic rate of contracting muscle, our muscular system produces a great deal of waste heat. Many small muscle contractions within the body produce our natural body heat. When we exert ourselves more than normal, the extra muscle contractions lead to a rise in body temperature and eventually to sweating.
Skeletal Muscles as Levers
- Skeletal muscles work together with bones and joints to form lever systems. The muscle acts as the effort force; the joint acts as the fulcrum; the bone that the muscle moves acts as the lever; and the object being moved acts as the load.
- There are three classes of levers, but the vast majority of the levers in the body are third class levers. A third class lever is a system in which the fulcrum is at the end of the lever and the effort is between the fulcrum and the load at the other end of the lever. The third class levers in the body serve to increase the distance moved by the load compared to the distance that the muscle contracts.
- The tradeoff for this increase in distance is that the force required to move the load must be greater than the mass of the load. For example, the biceps brachia of the arm pulls on the radius of the forearm, causing flexion at the elbow joint in a third class lever system. A very slight change in the length of the biceps causes a much larger movement of the forearm and hand, but the force applied by the biceps must be higher than the load moved by the muscle.
- MOTOR UNIT:
Nerve cells called motor neurons control the skeletal muscles. Each motor neuron controls several muscle cells in a group known as a motor unit. When a motor neuron receives a signal from the brain, it stimulates all of the muscles cells in its motor unit at the same time.
- The size of motor units varies throughout the body, depending on the function of a muscle. Muscles that perform fine movements—like those of the eyes or fingers—have very few muscle fibers in each motor unit to improve the precision of the brain’s control over these structures. Muscles that need a lot of strength to perform their function—like leg or arm muscles—have many muscle cells in each motor unit. One of the ways that the body can control the strength of each muscle is by determining how many motor units to activate for a given function. This explains why the same muscles that are used to pick up a pencil are also used to pick up a bowling ball
Muscles contract when stimulated by signals from their motor neurons. Motor neurons contact muscle cells at a point called the Neuromuscular Junction (NMJ). Motor neurons release neurotransmitter chemicals at the NMJ that bond to a special part of the sarcolemma known as the motor end plate. The motor end plate contains many ion channels that open in response to neurotransmitters and allow positive ions to enter the muscle fiber. The positive ions form an electrochemical gradient to form inside of the cell, which spreads throughout the sarcolemma and the T-tubules by opening even more ion channels.
CONTRACTION CYCLE :
- When the positive ions reach the sarcoplasmic reticulum, Ca2+ ions are released and allowed to flow into the myofibrils. Ca2+ ions bind to troponin, which causes the troponin molecule to change shape and move nearby molecules of tropomyosin. Tropomyosin is moved away from myosin binding sites on actin molecules, allowing actin and myosin to bind together.
- ATP molecules power myosin proteins in the thick filaments to bend and pull on actin molecules in the thin filaments. Myosin proteins act like oars on a boat, pulling the thin filaments closer to the center of a sarcomere. As the thin filaments are pulled together, the sarcomere shortens and contracts. Myofibrils of muscle fibers are made of many sarcomeres in a row, so that when all of the sarcomeres contract, the muscle cells shorten with a great force relative to their size.
- Muscles continue contraction as long as they are stimulated by a neurotransmitter. When a motor neuron stops the release of the neurotransmitter, the process of contraction reverses itself. Calcium returns to the sarcoplasmic reticulum; troponin and tropomyosin return to their resting positions; and actin and myosin are prevented from binding. Sarcomeres return to their elongated resting state once the force of myosin pulling on actin has stopped.
- Certain conditions or disorders, such as myoclonus, can affect the normal contraction of muscles. You can learn about musculoskeletal health problems in our section devoted to diseases and conditions. Also, learn more about advances in DNA health testing that help us understand the genetic risk of developing early-onset primary dystonia.
Types of Muscle Contraction :
- The strength of a muscle’s contraction can be controlled by two factors: the number of motor units involved in contraction and the amount of stimulus from the nervous system. A single nerve impulse of a motor neuron will cause a motor unit to contract briefly before relaxing. This small contraction is known as a twitch contraction. If the motor neuron provides several signals within a short period of time, the strength and duration of the muscle contraction increase. This phenomenon is known as temporal summation. If the motor neuron provides many nerve impulses in rapid succession, the muscle may enter the state of tetanus, or complete and lasting contraction. A muscle will remain in tetanus until the nerve signal rate slows or until the muscle becomes too fatigued to maintain tetanus.
Not all muscle contractions produce movement. Isometric contractions are light contractions that increase the tension in the muscle without exerting enough force to move a body part. When people tense their bodies due to stress, they are performing an isometric contraction. Holding an object still and maintaining posture are also the result of isometric contractions. A contraction that does produce movement is an isotonic contraction. Isotonic contractions are required to develop muscle mass through weight lifting.
Muscle tone is a natural condition in which a skeletal muscle stays partially contracted at all times. Muscle tone provides a slight tension on the muscle to prevent damage to the muscle and joints from sudden movements, and also helps to maintain the body’s posture. All muscles maintain some amount of muscle tone at all times, unless the muscle has been disconnected from the central nervous system due to nerve damage.
Functional Types of Skeletal Muscle Fibers
- Skeletal muscle fibers can be divided into two types based on how they produce and use energy: Type I and Type II.
- Type I fibers are very slow and deliberate in their contractions. They are very resistant to fatigue because they use aerobic respiration to produce energy from sugar. We find Type I fibers in muscles throughout the body for stamina and posture. Near the spine and neck regions, very high concentrations of Type I fibers hold the body up throughout the day.
Type II fibers are broken down into two subgroups: Type II A and Type II B.
Type II A fibers are faster and stronger than Type I fibers, but do not have as much endurance. Type II A fibers are found throughout the body, but especially in the legs where they work to support your body throughout a long day of walking and standing.
Type II B fibers are even faster and stronger than Type II A, but have even less endurance. Type II B fibers are also much lighter in color than Type I and Type II A due to their lack of myoglobin, an oxygen-storing pigment. We find Type II B fibers throughout the body, but particularly in the upper body where they give speed and strength to the arms and chest at the expense of stamina.
- Muscle Metabolism and Fatigue
- Muscles get their energy from different sources depending on the situation that the muscle is working in. Muscles use aerobic respiration when we call on them to produce a low to moderate level of force. Aerobic respiration requires oxygen to produce about 36-38 ATP molecules from a molecule of glucose. Aerobic respiration is very efficient, and can continue as long as a muscle receives adequate amounts of oxygen and glucose to keep contracting. When we use muscles to produce a high level of force, they become so tightly contracted that oxygen carrying blood cannot enter the muscle. This condition causes the muscle to create energy using lactic acid fermentation, a form of anaerobic respiration. Anaerobic respiration is much less efficient than aerobic respiration—only 2 ATP are produced for each molecule of glucose. Muscles quickly tire as they burn through their energy reserves under anaerobic respiration.
- To keep muscles working for a longer period of time, muscle fibers contain several important energy molecules. Myoglobin, a red pigment found in muscles, contains iron and stores oxygen in a manner similar to hemoglobin in the blood. The oxygen from myoglobin allows muscles to continue aerobic respiration in the absence of oxygen. Another chemical that helps to keep muscles working is creatine phosphate. Muscles use energy in the form of ATP, converting ATP to ADP to release its energy. Creatine phosphate donates its phosphate group to ADP to turn it back into ATP in order to provide extra energy to the muscle. Finally, muscle fibers contain energy-storing glycogen, a large macromolecule made of many linked glucoses. Active muscles break glucoses off of glycogen molecules to provide an internal fuel supply.
- When muscles run out of energy during either aerobic or anaerobic respiration, the muscle quickly tires and loses its ability to contract. This condition is known as muscle fatigue. A fatigued muscle contains very little or no oxygen, glucose or ATP, but instead has many waste products from respiration, like lactic acid and ADP. The body must take in extra oxygen after exertion to replace the oxygen that was stored in myoglobin in the muscle fiber as well as to power the aerobic respiration that will rebuild the energy supplies inside of the cell. Oxygen debt (or recovery oxygen uptake) is the name for the extra oxygen that the body must take in to restore the muscle cells to their resting state. This explains why you feel out of breath for a few minutes after a strenuous activity—your body is trying to restore itself to its normal stat.
The Muscle Groups And Their Actions
- The neck
-The motion of the neck is described in terms of rotation, flexion, extension, and side bending (i.e., the motion used to touch the ear to the shoulder). The direction of the action can be ipsilateral, which refers to movement in the direction of the contracting muscle, or contralateral, which refers to movement away from the side of the contracting muscle.
- Rotation is one of the most important actions of the cervical (neck) spine. Rotation is accomplished primarily by the sternocleidomastoid muscle, which bends the neck to the ipsilateral side and rotates the neck contralaterally. Together, the sternocleidomastoid muscles on both sides of the neck act to flex the neck and raise the sternum to assist in forced inhalation. The anterior and middle scalene muscles, which also are located at the sides of the neck, act ipsilaterally to rotate the neck, as well as to elevate the first rib. The splenius capitis and splenius cervicis, which are located in the back of the neck, work to rotate the head
2) Side bending also is an important action of the cervical spine. The sternocleidomastoid muscles are involved in cervical side bending. The posterior scalene muscles, located on the lower sides of the neck, ipsilaterally bend the neck to the side and elevate the second rib. The splenius capitis and splenius cervicis also assist in neck-side bending. The erector spinae muscles (iliocostalis, longissimus, and spinalis) are large, deep muscles that extend the length of the back. All three act to ipsilaterally side bend the neck. -
3)Neck flexion refers to the motion used to touch the chin to the chest. It is accomplished primarily by the sternocleidomastoid muscles, with assistance from the longus colli and the longus capitis, which are found in the front of the neck. Neck extension is the opposite of flexion and is accomplished by many of the same muscles that are used for other neck movements, including the splenius cervicis, splenius capitis, iliocostalis, longissimus, and spinalis muscles.
The back
The back contains the origins of many of the muscles that are involved in the movement of the neck and shoulders. In addition, the axial skeleton that runs vertically through the back protects the spinal cord, which innervates almost all the muscles in the body.
- Multiple muscles in the back function specifically in movements of the back. The erector spinae muscles, for example, extend the back (bend it backward) and side bend the back. The semispinalis dorsi and semispinalis capitis muscles also extend the back. The small muscles of the vertebrae (the multifidi and rotators) help rotate, extend, and side bend the back. The quadratus lumborum muscle in the lower back side bends the lumbar spine and aids in the inspiration of air through its stabilizing affects at its insertion at the 12th rib (the last of the floating ribs). The scapula (shoulder blade) is elevated by the trapezius muscle, which runs from the back of the neck to the middle of the back, by the rhomboid major and rhomboid minor muscles in the upper back, and by the levator scapulae muscle, which runs along the side and back of the neck.
- The shoulder
The shoulder is a complex ball-and-socket joint comprising the head of the humerus, the Clavicle (collarbone), and the scapula. The shoulder’s main motions are flexion, extension, abduction, adduction, internal rotation, and external rotation.
- Shoulder flexion is the movement of the shoulder in a forward motion. An example of shoulder flexion can be seen when reaching forward to grasp an object. That action is accomplished primarily by the combined actions of the deltoid muscle in the uppermost extent of the arm, the pectoralis major muscle in the chest, the coracobrachialis muscle on the inside of the upper arm, and the biceps brachii muscles on the front of the upper arm.
- Extension of the shoulder is opposite to flexion. Pure shoulder extension is the movement of the arm directly behind the body, as in receiving a baton in a relay race. That movement is accomplished by the actions of the deltoid muscle, the latissimus dorsi muscle in the back, the teres major muscle in the armpit area, and the triceps muscle in the back of the upper arm.The triceps, as the name suggests, consists of three heads that originate from different surfaces but share the same insertion at the olecranon process of the ulna (a bone in the forearm); the three heads together act to extend the elbow.
- Shoulder adduction and abduction serve to lower the arm toward and lift the arm away from the body, respectively. They can be visualized by picturing someone doing jumping jacks. Adduction is accomplished primarily by the pectoralis major, latissimus dorsi, teres major, triceps, and coracobrachialis. The deltoid and the supraspinatus, a muscle that runs along the scapula in the back, are the two main abductors of the shoulder.
- An example of external rotation of the shoulder is seen in a tennis backhand stroke. External rotation is attributed primarily to the deltoid, the teres minor in the armpit area, and the infraspinatus muscle, which covers the scapula. Internal rotation of the shoulder is the opposite of external rotation. An example is the shoulder movement that occurs when reaching
into a back pocket. That movement is achieved through the coordinated action of the pectoralis major, latissimus dorsi, deltoid, teres major, and subscapularis muscles. (The subscapularis is a deep muscle situated on the anterior, or front-facing, surface of the scapula.)
- The teres minor, subscapularis, supraspinatus, and infraspinatus muscles together form the rotator cuff, which stabilizes the humeral head (the ball portion of the ball-and-socket shoulder joint). The muscles of the rotator cuff are common sites of injury in adults, particularly among people who perform overhead motions repeatedly (e.g., throwing a baseball or painting a ceiling). Several of the rotator cuff muscles have tendons that run under the acromion, a bony prominence at the distal end of the scapula. (The term distal describes a relative position away from the centre of the body; it often is contrasted with the term proximal, which describes a relative position near to the centre of the body.) The position of the tendons and of the subacromial bursae (fluid-filled sacs located beneath the acromion)
leaves them vulnerable to compression and pinching, which can result in an injury known as shoulder impingement syndrome.
- The arm
In addition to aiding the movement of the shoulder, the muscles of the upper arm produce various movements of the forearm. For example, the primary muscles involved in forearm flexion, in which the angle formed at the elbow becomes smaller (i.e., the hand moves closer to the shoulder), are the biceps brachii, the brachialis (situated beneath the biceps brachii
in the upper arm), and the brachioradialis (the origin of which is on the humerus). Minor contributions to forearm flexion are provided by the coracobrachialis and by flexor muscles situated in the anterior compartment of the forearm (the palm side of the forearm; also known as the flexor compartment), including the pronator teres, the flexorcarpi radialis, the flexor digitorum superficialis, the palmaris longus, and the flexor carpi ulnaris.
- Extension of the forearm increases the angle at the elbow, moving the hand away from the shoulder. That action is accomplished primarily by the triceps brachii. Other muscles that make minor contributions to forearm extension include the extensor muscles of the posterior compartment of the forearm (the side of the forearm that is contiguous with the back of the hand; also known as the extensor compartment), including the extensor carpi radialis longus, the extensor carpi radialis brevis, the extensor digitorum, the extensor carpi ulnaris, and the anconeus.
- The wrist
Wrist flexion refers to the movement of the wrist that draws the palm of the hand downward. That action is carried out by the flexor carpi radialis, the flexor carpi ulnaris, the flexor digitorum superficialis, the flexor digitorum profundus, and the flexor pollicis longus.
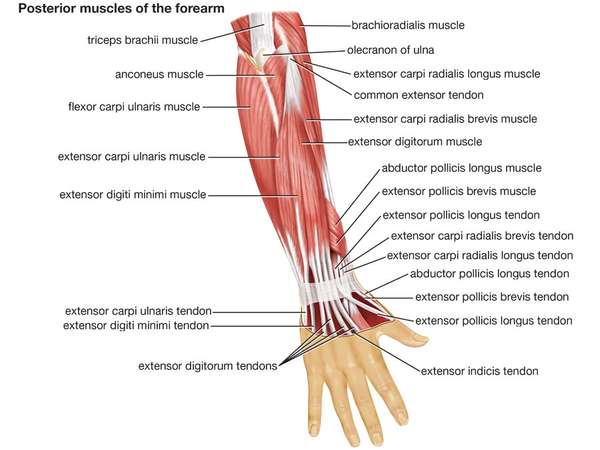
- Wrist extension, by contrast, shortens the angle at the back of the wrist. The muscles responsible for that action are the extensor carpi radialis longus and the extensor carpi radialis brevis, which also abduct the hand at the wrist (move the hand in the direction of the thumb, or first digit); the extensor digitorum, which also extends the index to the little finger (the second to fifth digits); the extensor digiti minimi, which also extends the little FInger and adducts the hand (moves the hand in the direction of the little finger); and the extensor carpi ulnaris, which also adducts the hand. Other small muscles that cross the wrist joint may add to wrist extension, but they do so to only a small degree.
- Wrist supination is the rotation of the wrist that brings the palm facing up. The supinator muscle in the posterior compartment acts to supinate the forearm. The biceps brachii also adds to supination. Pronation is the opposing action, in which the wrist is rotated so that the palm is facing down. The pronator quadratus, a deep muscle in the anterior compartment, along with the pronator teres, pronates the forearm.
The hand
- The hand is a complex structure that is involved in fine motor coordination and complex task performance. Its muscles generally are small and extensively innervated. Even simple actions, such as typing on a keyboard, require a multitude of precise movements to be carried out by the hand muscles. Because of that complexity, the following paragraphs cover only the primary action of each hand muscle.
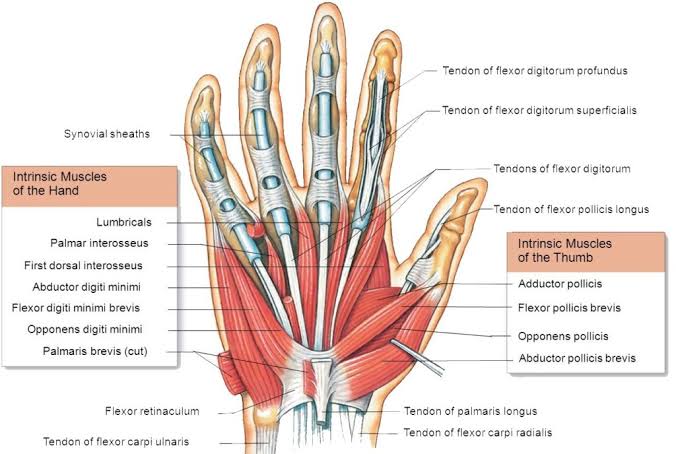
- Although several of the muscles that move the hand have their origins in the forearm, there are many small muscles of the hand that have both their origin and their insertion within the hand. Those are referred to as the intrinsic muscles of the hand. They include the palmaris brevis, which assists with grip; the umbricals, which flex the MCP joints and extend the interphalangeal joints (IPs; the joints between the phalanges) of the fingers; the palmar interossei, which adduct the fingers toward the middle finger (the third digit); and the dorsal interossei, which abduct the fingers away from the middle finger. All the interossei flex the MCP joints and extend the IP joints.
- The thenar eminence is located on the palm side of the base of the thumb and is composed of three muscles, the abductor pollicis brevis, the flexor pollicis brevis, and the opponens pollicis, all of which are innervated by the median nerve. The abductor pollicis brevis abducts the thumb; the flexor pollicis brevis flexes the MCP joint of the thumb; and the opponens pollicis acts to oppose the thumb to the other fingers. The adductor pollicis, which
is not part of the thenar eminence, acts to adduct the thumb.
- The hypothenar enimence is located on the palm side of the hand below the little finger. It contains three muscles that are innervated by the deep branch of the ulnar nerve. The abductor digiti minimi abducts the little finger. The flexor digiti minimi flexes the little finger. The opponens digiti minimi opposes the little finger with the thumb
- The abdomen
There are three muscular layers of the abdominal wall, with a fourth layer in the middle anterior region. The fourth layer in the midregion is the rectus abdominis, which has vertically running muscle fibres that flex the trunk and stabilize the pelvis. To either side of the rectus abdominis are the other three layers of abdominal muscles. The deepest of those layers is the transversus abdominis, which has fibres that run perpendicular to the rectus abdominus; the transversus abdominis acts to compress and support the abdomen and provides static core stabilization. The internal oblique layers run upward and forward from the sides of the abdomen, and the external oblique layers, which form the outermost muscle layers of the abdomen, run downward and forward. The internal oblique layers act in conjunction with the external oblique on the opposite side of the body to flex and rotate the trunk toward the side of the contracting internal oblique (“same-side rotator”)
The hip
The hip joint is a complex weight-bearing ball-and-socket joint that can sustain considerable load. The socket of the joint is relatively deep, allowing for stability but sacrificing some degree in range of motion. The movements described in this section include flexion, extension, abduction, and adduction.
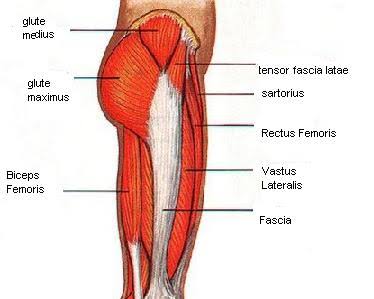
Hip flexion is the hip motion that brings the knee toward the chest. The major muscles of hip flexion include the iliopsoas, which is made up of the psoas major, psoas minor, and iliacus. Together, those muscles act mainly to flex the hip, but they also contribute to abdominal flexion and hip stabilization. Other hip flexors include the sartorius, the rectus femoris, the pectineus, and the gracilis. The sartorius also contributes to external hip rotation and knee extension and abduction, and the rectus femoris also acts in knee extension. The pectineus is also involved in hip adduction and internal rotation.
Hip extension is accomplished primarily by the muscles of the posterior thigh and buttocks, which when contracted serve to move the thigh from a flexed position toward the midline of the body or the trunk of the body from a bent position toward a more-erect posture. Hip extension is accomplished mostly by the gluteus maximus, the biceps femoris (which is divided into two heads, the long head and the short head), the semitendinosus, and the semimembranosus. A minor contribution is also provided by the adductor magnus and other small pelvic muscles.
The movement of adduction is used to describe a direction of limb motion that serves to take the limb from a lateral position to its more-axial alignment. During a jumping-jack exercise, for example, abduction of the leg occurs when it is moved away from the midline and adduction when it is moved back toward the midline. The main abductors of the hip are the gluteus medius, gluteus minimus, and tensor fascia lata. Those three muscles also serve to internally rotate the thigh in an extended position and externally rotate the thigh in a flexed position. Another minor contributor is the piriformis. The main hip adductors are the adductor magnus,
he adductor brevis, and the adductor longus. A minor contribution to hip adduction is performed by the pectineus and the gracilis.
The upper leg and knee
Extension of the knee is accomplished by a group of muscles collectively referred to as the quadriceps femoris, which increases the angle of the knee, bringing the lower leg into a straight position. Knee extension is used in the forward, and swing phase of the gait and is integral in movements such as kicking. The quadriceps femoris group includes the vastus medius,vastus lateralis, vastus intermedius, and rectus femoris. A minor contribution to knee extension is provided by the sartorius.
Knee flexion refers to the bending of the knee from a straight position. The muscles that perform that action oppose those of knee extension and are generally referred to as the hamstring muscles. The hamstring muscles are situated in the back of the thigh and include the biceps femoris, the semitendinosus, and the semimembranosus. Small contributions to knee flexion are made by the gastrocnemius muscle in the back of the calf and by several small muscles that cross the knee joint posteriorly.
The lower leg and foot
the muscles of the lower leg and foot are complex and work in many planes. Their actions depend on whether the person is bearing weight, as well as on the position of the foot. The following paragraphs provide a brief overview of the actions of the muscles of the lower leg and foot.
Dorsiflexion refers to ankle flexion in the direction of the dorsum, or anterior surface of the foot (the surface of the foot viewed from above). Dorsiflexion is accomplished by several muscles, including the tibialis anterior, which in addition to dorsiflexion also inverts the foot (tilts the foot toward the midline), stabilizes the foot when striking the ground, and locks the ankle when kicking. The extensor digitorum longus (EDL) also acts in dorsiflexion and functions to extend the last four toes. In addition to the EDL, some individuals also have a muscle called the peroneus tertius (fibularis tertius), which participates to a limited extent in dorsiflexion and eversion of the foot (tilting of the foot away from the midline). The extensor hallucis longus primarily acts in big toe (hallux) dorsiflexion, but it also acts
to dorsiflex, as well as weakly invert, the ankle.
Plantarflexion refers to flexion of the ankle in the direction of the sole of the foot. That is most easily demonstrated by having a person stand on his or her toes. The majority of ankle plantarflexion is performed by the large calf musculature, including the gastrocnemius and the soleus, which lies just behind the gastrocnemius. It is generally accepted that those are two
distinct muscles; however, there is some debate as to whether the gastrocnemius and the soleus are two parts of the same muscle.
Other muscles of the lower leg and foot include the plantaris, which runs obliquely between gastrocnemius and the soleus; the flexor hallucis longus, which contributes to ankle flexion but is involved primarily in big toe flexion; the flexor digitorum longus, which also flexes the second to fifth toes; the peroneus longus, which flexes the ankle and everts the foot; and the peroneus brevis, which is involved in plantarflexion and eversion of the foot.
Intrinsic muscles of the foot arise in the foot and do not cross the ankle joint. Hence, their action is confined to the foot. The intrinsic muscles of the foot include the abductor hallucis, which abducts the big toe; the flexor digitorum brevis, which flexes the second to fifth toes; the abductor digiti minimi, which abducts and flexes the fifth toe; the quadratus plantae, which assists in toe flexion; the lumbricals, which flex the metatarsophalangeal (MTP) joints and extend the distal IP and proximal IP joints of the toes; the flexor hallucis brevis, which flexes the big toe; and the adductor hallucis, which flexes and contracts the big toe. The adductor hallucis has two heads, the oblique head and the transverse head, which share an insertion on the lateral (outer) side of the base of the proximal phalanx of the big toe. The oblique head arises from the base of the second to fourth metatarsal bones, and the transverse head arises from the ligaments of the MTP joints of the third to fifth toes. The flexor digiti minimi brevis extends and adducts the fifth toe. The dorsal interossei abduct the toes, and the plantar interossei adduct the toes.
Muscle Strength
Introduction
An assessment of muscle strength is typically performed as part of a patient’s objective assessment. This should assist the physiotherapist’s clinical reasoning and enable them to reason an appropriate point to begin strengthening rehabilitation from. Muscle strength can be assessed by a number of methods: manually, functionally, or mechanically.
The Oxford Scale
The Oxford scale is commonly used by physiotherapists to manually assess muscle strength. According to the Oxford scale, muscle strength is graded 0 to 5. The grades are summarized
below:
1)Flicker of movement
2)Through full range actively with gravity counterbalanced
3)Through full range actively against gravity
4)Through full range actively against some resistance
5)Through full range actively against strong resistance
There are a number of limitations to the usefulness of the Oxford scale.
These include:
1)Poor functional relevance;
2)Non-linearity i.e. the difference between grades 3 and 4 is not necessarily the same as the difference between grades 4 and 5;
3)A patient’s variability over time i.e, alternating between grades due to fatigue;
4)Intra-rater reliability;
5)Only assesses muscles when contracting concentrically; The difficulty of applying the Oxford Scale to all patient’s in clinical practice (so that strength is rarely assessed throughout full range as many patients assessed by physiotherapists do not possess full range due to their respective pathology.
Due to these shortcomings, physiotherapists commonly use modified versions of the Oxford scale
in clinical practice.
Types of Muscle Contractions: Isotonic and Isometric
1)Isotonic contractions generate force by changing the length of the muscle and can be concentric contractions or eccentric contractions.
2)A concentric contraction causes muscles to shorten, thereby generating force.
3)Eccentric contractions cause muscles to elongate in response to a greater opposing force.
4)Isometric contractions generate force without changing the length of the muscle.
- Isometric: A muscular contraction in which the length of the muscle does not change.
- isotonic: A muscular contraction in which the length of the muscle changes.
- eccentric: An isotonic contraction where the muscle lengthens.
- concentric: An isotonic contraction where the muscle shortens.
A muscle fiber generates tension through actin and myosin cross-bridge cycling. While under tension, the muscle may lengthen, shorten, or remain the same. Although the term contraction implies shortening, when referring to the muscular system, it means the generation of tension within a muscle fiber. Several types of muscle contractions occur and are defined by the changes in the length of the muscle during contraction.
Isotonic Contractions
Isotonic contractions maintain constant tension in the muscle as the muscle changes length. Isotonic muscle contractions can be either concentric or eccentric.
Concentric Contractions
A concentric contraction is a type of muscle contraction in which the muscles shorten while generating force, overcoming resistance. For example, when lifting a heavy weight, a concentric contraction of the biceps would cause the arm to bend at the elbow, lifting the weight towards the shoulder. Cross-bridge cycling occurs, shortening the sarcomere, muscle
fiber, and muscle.
Eccentric Contractions
An eccentric contraction results in the elongation of a muscle while the muscle is still generating force; in effect, resistance is greater than force generated. Eccentric contractions can be both voluntary and involuntary. For example, a voluntary eccentric contraction would be the controlled lowering of the heavy weight raised during the above concentric contraction. An involuntary eccentric contraction may occur when a weight is too great for a muscle to bear and so it is slowly lowered while under tension. Cross-bridge cycling occurs even though the sarcomere, muscle fiber, and muscle are lengthening, controlling the extension of the muscle.
Types of Muscle Contraction: An isotonic concentric contraction results in the muscle shortening, an isotonic eccentric contraction results in the muscle lengthening. During an isometric contraction the muscle is under tension but neither shortens nor lengthens.
Isometric Contractions
In contrast to isotonic contractions, isometric contractions generate force without changing the length of the muscle, common in the muscles of the hand and forearm responsible for grip. Using the above example, the muscle contraction required to grip but not move a heavy object
prior to lifting would be isometric. Isometric contractions are frequently used to maintain posture.
Isometric contractions are sometimes described as yielding or overcoming.
Yielding
A yielding contraction occurs when a muscle contraction is opposed by resistance. For example, when holding a heavy weight steady, neither raising nor lowering it.
Overcoming
An overcoming contraction occurs when a muscle contraction is opposed by an immovable object, such as the contraction generated in the muscles when pushing against a wall.
In both instances, cross-bridge cycling is maintaining tension in the muscle; the sarcomere, muscle fibers, and muscle are not changing length.
3 Comments